ENERGY DENSITY: GETTING TO LONGER DRIVING RANGES
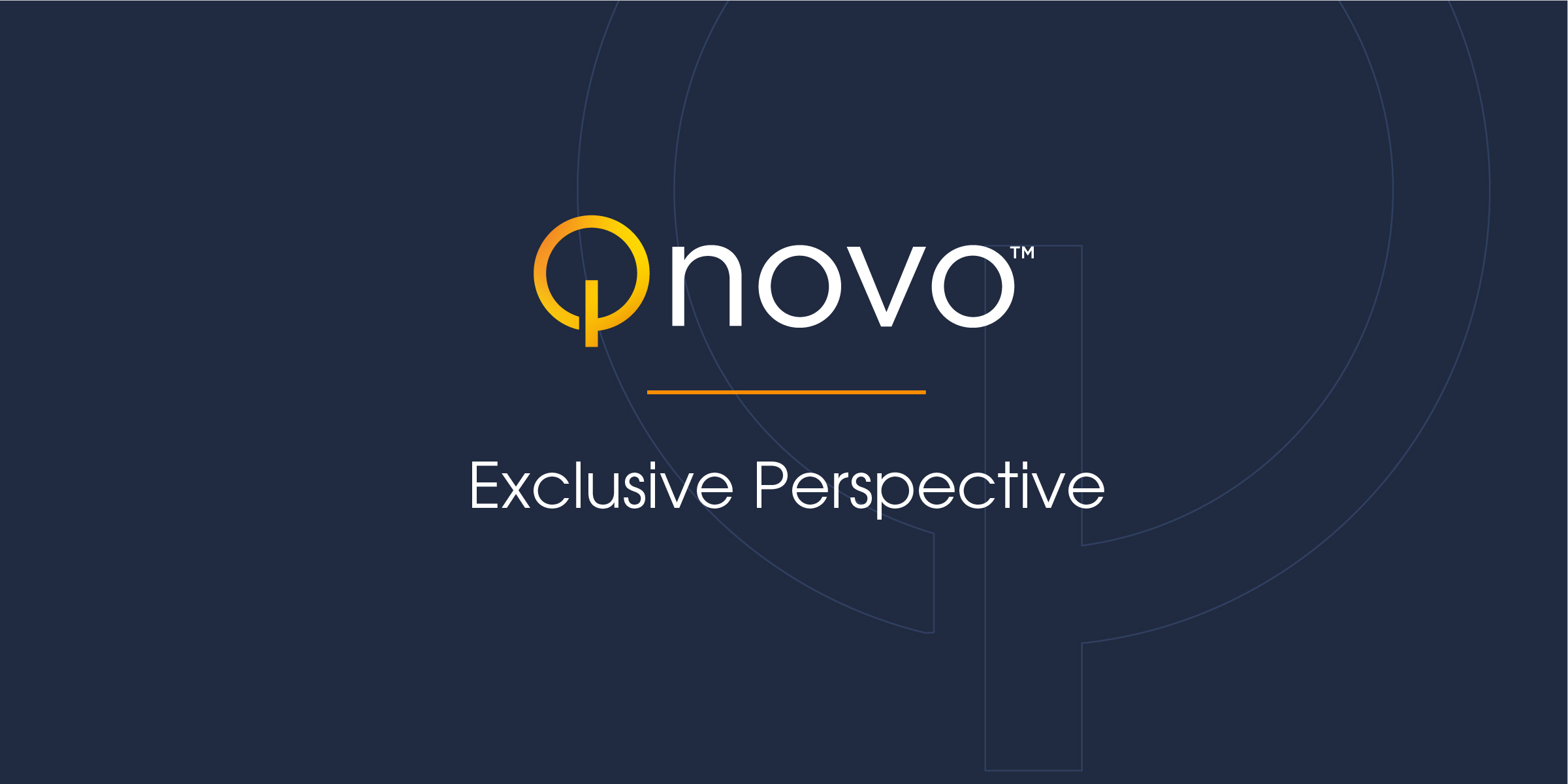
Three factors contribute to the mass penetration of electric vehicles:
- Driving range that eliminates range anxiety;
- Lower cost batteries for affordable vehicles;
- Availability of fast charging.
A common denominator is the battery’s energy density: it is the amount of electrical energy per unit volume (or per unit weight) that a rechargeable battery can store. Energy is measured in units of kWh. Hence energy density is in units of Wh per liter (Wh/l), or Wh per kg (Wh/kg). State-of-the-art energy density figures for lithium-ion batteries stand today near 700 Wh/l and 300 Wh/kg.
Driving range is a direct function of the total energy capacity of the battery. The battery in a Tesla Model S with a driving range of 400 miles can hold 100 kWh. Whereas the battery in a Model 3 with a driving range of 322 miles can hold only 75 kWh.
Energy density determines the size and weight of the battery. Both Model S and Model 3 vehicles use similar energy density, hence one would expect the battery in the Model S to be 25% bigger. And they are, approximately!
The second consequence of energy density is cost. The price of lithium-ion batteries is measured in units of dollars per kWh. This benchmark price stood in 2019 near $150 per kWh for the entire pack, i.e., a fully assembled battery including cells, wiring, electronics…etc. The cost implications are complex and depend on the scale of manufacturing but history over the past decade has shown that, in the long term, cost does trend down as energy density rises.
The third consequence of energy density impacts the wide availability of fast charging. An important note here: this means that the charging infrastructure needs to be upgraded to fast charging stations, and that EV batteries must be designed to safely accept the fast charging rate from these new stations. It is the latter part that is the subject relating to energy density.
Generally, there is a natural design tension between increasing energy density and fast charging. Increasing energy density means more tightly packed electrode layers inside the battery. But this packing makes it more challenging for the lithium ions to move “faster” during fast charging. This challenge can be mitigated with balancing the battery structural design and with intelligent algorithms to manage the battery charging.
So how do battery manufacturers raise the energy density? And what challenges do they face? Let’s explore a little.
Basic physics tell us that the stored energy in the battery takes the form of electrical charge; here it is strictly the positively charged lithium ion (Li+). Therefore, increasing energy density means holding more lithium ions in the space occupied by the battery. This space consists of the following materials:
- The positive electrode (cathode);
- The negative electrode (anode);
- And everything else, which includes connectors and insulators.
The two electrodes are the materials that can hold lithium ions – the ions intercalate physically within the fabric of the electrodes, like raisins embedded within a raisin bread. The rest are there to support the function of the battery but they do not hold electrical charge. So in some sense, everything other than the two electrodes is “overhead.”
For years, battery manufacturers increased energy density by minimizing this overhead. They reduced the thickness of its layers and made designs that minimized the amount of overhead material. By the middle of the past decade, these benefits were pretty much exhausted. So battery designs began to raise the electrical voltage across the battery. Since energy is the amount of charge multiplied by voltage, it was easier for manufacturers to play with the voltage knob. This method is also reaching its limit.
Now, battery manufacturers are facing the only remaining option: how to increase the number of ions that can be held within the two electrodes. This is precisely what large battery makers in Asia and the multitude of global startups are trying to accomplish.
Let’s start with the first electrode. The cathode in your smartphone’s battery is made of a material called lithium-cobalt-oxide (LCO). Its capacity to hold ions has already maxed out. Another material commonly used in China, lithium-iron-phosphate (LFP), is safer than the fire-prone cobalt-laden LCO, but at the expense of much lower energy density.
Modern EV batteries use two new types of composite cathode materials. Tesla batteries, made by Panasonic, use a cathode material called nickel-cobalt-aluminum-oxide (NCA). Batteries in EVs made for GM, VW, Daimler, BMW and several other car makers use a composite material made of nickel-cobalt-manganese-oxide (NCM). Both NCM and NCA are capable of holding more ions within their fabric – about 15% to 25% more, respectively. Some forms of NCM reduce the cobalt content by replacing it with nickel. Cobalt is a very expensive metal with primary mines in Africa.
The second electrode, the anode, is typically made of carbon, specifically, graphite…it is similar to the graphite in a pencil lead. It was Akira Yoshino’s discovery of graphite as an anode material in the 1980s that paved the way to mass manufacturing of lithium-ion batteries. For his work, he earned the 2019 Nobel Prize in Chemistry.
Graphite anodes are quite porous allowing the lithium ions to intercalate between the carbon atoms. This is how the electrical charge is held within the battery during charging. For each lithium ion, it takes six carbon atoms to store one unit of electrical charge.
As an anode material, graphite has reached its limits. Enter silicon with a far higher capacity to store lithium ions in its fabric. One silicon atom can hold almost 4 lithium ions — or four units of charge (to be exact, 4 silicon atoms to 15 lithium ions). That’s a significant jump over graphite. But — there is always a but — the extra lithium ions cause the silicon to physically expand and swell, placing enormous mechanical stresses on its crystalline structure and ultimately causing its fracture. So much of the on-going material development is on methods to manufacture silicon-graphite composites that can hold more charge but be more resilient to mechanical failure.
The combination of new cathode materials with silicon-graphite composite anodes promise to deliver energy densities around 900 ~ 1,000 Wh/l. Yet, the vast majority of lithium ion batteries continue to ship today with graphite anodes highlighting the difficulties and the long durations needed for bringing new materials to market.